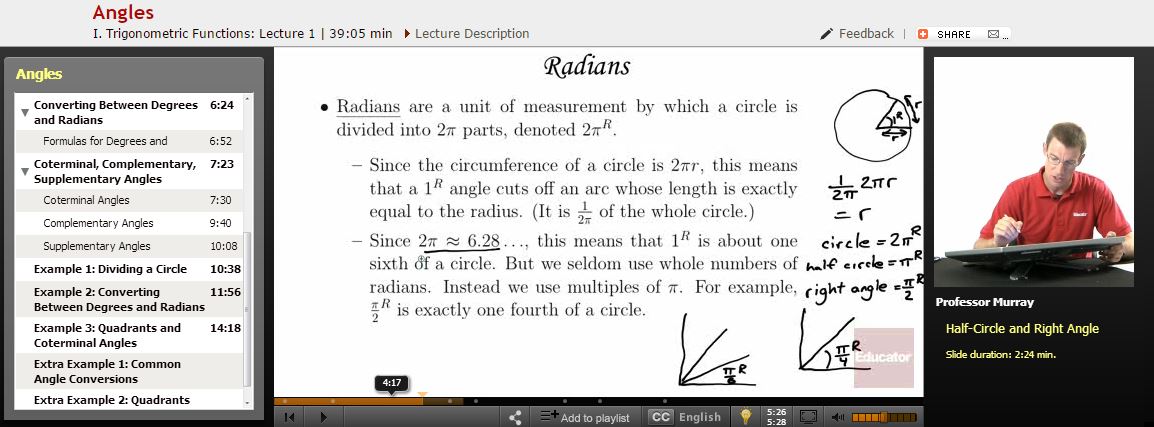
There was a time when Chemistry was kind
Lewis dots formed bonds
With their orbitals aligning!
There was a time…
Then it all went wrong.
We all dreamed a dream, in time gone by… when atoms only needed eight electrons in their outer shell. If you put them together with other atoms, the molecules were stable. Simple. Pristine. Like the summers of years past.
Then, you move on to college, and everything falls apart. All those beautiful Lewis Dot diagrams become confusing molecular orbitals.
It’s no longer enough to know which electrons will be shared. You have to know how they’ll be shared, and where, and at what energy to know if the molecule will be stable. It can be a harsh awakening.
But there is hope! Once you understand how molecular orbital theories work, the universe of Chemistry will open. You’ll know how the molecule might react, and maybe even what wavelengths of light it absorbs.
It may not be simple, but it gives us powerful tools to understand what’s happening at a molecular level.
From Lewis Dot Diagrams to Molecular Orbitals
Let’s take your basic methane molecule, CH4. In a Lewis Dot Diagram, it would look like this:
Each pair of electrons forms a bond, which then try to get as far away from each other as possible (VSEPR theory), forming a tetrahedron.
But the electrons aren’t really sitting there in a straight line between the atoms. We know this from our knowledge of atomic orbitals. In a hydrogen atom, the electrons are sitting in a spherical s-orbital. In a carbon atom, they’re sitting in an s-orbital and three p-orbitals that look like dumbbells pointed along the x-, y-, and z-axes.
How do those electron clouds merge in real-world atoms? What do their final forms look like?
Molecular Bonding: Shared Electron Clouds
Here’s what happens:
S-orbitals can combine with other s-orbitals to form a sigma bonding orbital and a sigma* antibonding orbital, like this:
(there is no electron density at the node of the sigma* orbital, so you can imagine that these orbitals are pretty unstable and high-energy).
P orbitals combine to form pi bonding orbitals and pi* antibonding orbitals that look like this:
(same goes for the pi* orbital—high energy, and any electrons in it will make the molecule more unstable).
It’s all about the energies
Most of the chemistry of compounds is determined by which molecular orbitals are stable and full and which ones are empty and reactive. Their energies determine which other elements they can react with and even what wavelengths of light they can absorb.
Molecular Orbital Diagrams are used to represent these energies.
Basic molecular orbital diagrams
Each individual element’s electron configuration is depicted on the sides. Those orbitals come together in the center to form molecular orbitals of different energies. Bonding orbitals are lower in energy than anti-bonding orbitals, so they go lower down on the energy diagram. Sigma orbitals are lower energy than pi orbitals, while sigma* orbitals are higher energy than pi*.
Electrons in bonding orbitals stabilize and lower the energy of the molecule, whereas electrons in the antibonding orbital are destabilizing. As long as there are more bonding electrons than antibonding ones, the molecule will most likely be stable.
These diagrams get a little more complicated when more atoms are involved or atoms of differing energy levels are involved. Take CH4, for instance. In highschool, when the world was a song and that song was exciting, you probably learned that the s-orbital and three p-orbitals hybridize to form four identical sp3-hybridized orbitals that can then bond with the hydrogen s-orbitals. But ionization energy evidence points to a molecular orbital explanation, where the s-orbitals and p-orbitals form separate bonds:
One hydrogen s-orbitals gets pushed down in energy (stabilized) and bonds with the carbon s-orbital. The other three hydrogens bond with the p-orbitals. Only bonding orbitals are filled (no anti-bonding orbitals), so the molecule is very stable.
Why does it matter?
Orbitals matter because the energy levels dictate which atoms are likely to bond and whether they will be stable. They also tell you what wavelengths of light the molecule can absorb. When light (or another kind of radiation) hits a molecule, an electron gets promoted from a filled orbital to an empty one. In conjugated molecules, the antibonding orbitals can become more stable and cause the molecule to absorb visible light, making the material appear colored. This is how dyes work.
[box type=”success” align=”” class=”” width=””]It is easy to get lost in molecular orbital diagrams, but it’s important to remember what they do and the basic principles that govern them. Sit down with your professor if needed, or take a look at our lecture on Structures and Properties of Organic Molecules at Educator.com if you’re stuck. Molecular orbitals form the basis of a lot of the organic chemistry you’ll be doing, so it’s important to get a solid grasp on how they operate![/box]