
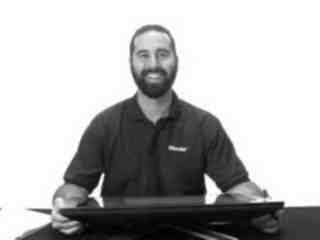
Michael Philips
DNA Replication
Slide Duration:Table of Contents
Section 1: The Beginnings of Molecular Biology
Biochemistry Review: Importance of Chemical Bonds
53m 29s
- Intro0:00
- Lesson Overview0:14
- Chemical Bonds0:41
- Attractive Forces That Hold Atoms Together0:44
- Types of Bonds0:56
- Covalent Bonds1:34
- Valence Number1:58
- H O N C P S Example2:50
- Polar Bonds7:23
- Non-Polar Bond8:46
- Non-Covalent Bonds9:46
- Ionic Bonds10:25
- Hydrogen Bonds10:52
- Hydrophobic Interactions11:34
- Van Der Waals Forces11:58
- Example 112:51
- Properties of Water18:27
- Polar Molecule13:34
- H-bonding Between Water H20 Molecules19:29
- Hydrophobic Interactions20:30
- Chemical Reactions and Free Energy22:52
- Transition State23:00
- What Affect the Rate23:27
- Forward and Reserve Reactions Occur Simultaneously But at Different Rate23:51
- Equilibrium State24:29
- Equilibrium Constant25:18
- Example 226:16
- Chemical Reactions and Free Energy27:49
- Activation Energy28:00
- Energy Barrier28:22
- Enzymes Accelerate Reactions by Decreasing the Activation Energy29:04
- Enzymes Do Not Affect the Reaction Equilibrium or the Change in Free Energy29:22
- Gibbs Free Energy Change30:50
- Spontaneity31:18
- Gibbs Free Energy Change Determines Final Concentrations of Reactants34:36
- Endodermic vs. Exothermic Graph35:00
- Example 338:46
- Properties of DNA39:37
- Antiparallel Orientation40:29
- Purine Bases Always Pairs Pyrimidine Bases41:15
- Structure Images42:36
- A, B, Z Forms43:33
- Major and Minor Grooves44:09
- Hydrogen Bonding and Hydrophobic Interactions Hold the Two Strands Together44:39
- Denaturation and Renaturation of DNA44:56
- Ways to Denature dsDNA45:28
- Renature When Environment is Brought Back to Normal46:05
- Hyperchromiicity46:36
- Absorbs UV Light47:01
- Spectrophotometer48:01
- Graph Example?49:05
- Example 451:02
Mendelian Genetics & Foundational Experiments
1h 9m 27s
- Intro0:00
- Lesson Overview0:22
- Gregor Johann Mendel1:01
- Was a Biologist and Botanist1:14
- Published Seminal Paper on Hybridization and Inheritance in the Pea Plant1:20
- Results Criticized1:28
- Father of Modern Genetics1:59
- Mendel’s Laws2:19
- 1st Law: Principle of Independent Segregation of Alleles2:27
- 2nd Law: Principle of Independent Assortment of Genes2:34
- Principle of Independent Segregation (of Alleles)2:41
- True Breeding Lines / Homozygous2:42
- Individuals Phenotypes Determined by Genes3:15
- Alleles3:37
- Alleles Can Be Dominant or Recessive3:50
- Genotypes Can be Experimentally Determined by Mating and Analyzing the Progeny5:36
- Individual Alleles Segregate Independently Into Gametes5:55
- Example 16:18
- Principle of Independent Segregation (of Alleles)16:11
- Individual Genes Sort Independently Into Gametes16:22
- Each Gamete Receives One Allele of Each Gene: 50/50 Chance16:46
- Genes Act Independently to Determine Unrelated Phenotypes16:57
- Example: Punnett Square17:15
- Example 221:36
- The Chromosomal Theory of Inheritance30:41
- Walter S Sutton Linked Cytological Studies with Mendels Work31:02
- Diploid Cells Have Two Morphologically Similar Sets of Chromosomes and Each Haploid Gamete Receives One Set31:17
- Genes Are on Chromosome31:33
- Gene for Seed Color’s on a Different Chromosome Than Gene for Seed Texture31:44
- Gene Linkage31:55
- Mendel’s 2nd Law31:57
- Genes Said to Be Linked To Each Other32:09
- Linkage Between Genes32:29
- Linkage is Never 100% Complete32:41
- Genes are Found on Chromosomes33:00
- Thomas Hunt Morgan and Drosophila Melanogaster33:01
- Mutation Linked to X Chromosome33:15
- Linkage of White Gene33:23
- Eye Color of Progeny Depended on Sex of Parent33:34
- Y Chromosome Does Not Carry Copy of White Gene33:44
- X Linked Genes, Allele is Expressed in Males33:56
- Example34:11
- Example 335:52
- Discovery of the Genetic Material of the Cell41:52
- Transforming Principle42:44
- Experiment with Streptococcus Pneumoniae42:55
- Beadle and Tatum Proposed Genes Direct the Synthesis of Enzymes45:15
- One Gene One Enzyme Hypothesis45:46
- One Gene One Polypeptide Theory45:52
- Showing the Transforming Material was DNA46:14
- Did This by Fractionating Heat-Killed “S” Strains into DNA, RNA, and Protein46:32
- Result: Only the DNA Fraction Could Transform47:15
- Leven: Tetranucleotide Hypothesis48:00
- Chargaff Showed This Was Not the Case48:48
- Chargaff: DNA of Different Species Have Different Nucleotide Composition49:02
- Hershey and Chase: DNA is the Genetic Material50:02
- Incorporate Sulfur into Protein and Phosphorous into DNA51:12
- Results: Phosphorase Entered Bacteria and Progeny Phage, But no Sulfur53:11
- Rosalind Franklin’s “Photo 51” Showing the Diffraction Pattern of DNA53:50
- Watson and Crick: Double Helical Structure of DNA54:57
- Example 456:56
- Discovery of the Genetic Material of the Cell58:09
- Kornberg: DNA Polymerase I58:10
- Three Postulated Methods of DNA Replication59:22
- Meselson and Stahl: DNA Replication is Semi-Conservative1:00:21
- How DNA Was Made Denser1:00:52
- Discovery of RNA1:03:32
- Ribosomal RNA1:03:48
- Transfer RNA1:04:00
- Messenger RNA1:04:30
- The Central Dogma of Molecular Biology1:04:49
- DNA and Replication1:05:08
- DNA and Transcription = RNA1:05:26
- RNA and Translation = Protein1:05:41
- Reverse Transcription1:06:08
- Cracking the Genetic Code1:06:58
- What is the Genetic Code?1:07:04
- Nirenberg Discovered the First DNA Triplet That Would Make an Amino Acid1:07:16
- Code Finished in 1966 and There Are 64 Possibilities or Triplet Repeats/ Codons1:07:54
- Degeneracy of the Code1:08:53
Section 2: Structure of Macromolecules
Structure of Proteins
49m 44s
- Intro0:00
- Lesson Overview0:10
- Amino Acids0:47
- Structure0:55
- Acid Association Constant1:55
- Amino Acids Make Up Proteins2:15
- Table of 21 Amino Acid Found in Proteins3:34
- Ionization5:55
- Cation6:08
- Zwitterion7:51
- Anion9:15
- Example 110:53
- Amino Acids13:11
- L Alpha Amino Acids13:19
- Only L Amino Acids Become Incorporated into Proteins13:28
- Example 213:46
- Amino Acids18:20
- Non-Polar18:41
- Polar18:58
- Hydroxyl19:52
- Sulfhydryl20:21
- Glycoproteins20:41
- Pyrrolidine21:30
- Peptide (Amide) Bonds22:18
- Levels of Organization23:35
- Primary Structure23:54
- Secondary Structure24:22
- Tertiary Structure24:58
- Quaternary Structure25:27
- Primary Structure: Specific Amino Acid Sequence25:54
- Example 327:30
- Levels of Organization29:31
- Secondary Structure: Local 3D29:32
- Example 430:37
- Levels of Organization32:59
- Tertiary Structure: Total 3D Structure of Protein33:00
- Quaternary Structure: More Than One Subunit34:14
- Example 534:52
- Protein Folding37:04
- Post-Translational Modifications38:21
- Can Alter a Protein After It Leaves the Ribosome38:33
- Regulate Activity, Localization and Interaction with Other Molecules38:52
- Common Types of PTM39:08
- Protein Classification40:22
- Ligand Binding, Enzyme, DNA or RNA Binding40:36
- All Other Functions40:53
- Some Functions: Contraction, Transport, Hormones, Storage41:34
- Enzymes as Biological Catalysts41:58
- Most Metabolic Processes Require Catalysts42:00
- Most Biological Catalysts Are Proteins43:13
- Enzymes Have Specificity of Reactants43:33
- Enzymes Have an Optimum pH and Temperature44:31
- Example 645:08
Structure of Nucleic Acids
1h 2m 10s
- Intro0:00
- Lesson Overview0:06
- Nucleic Acids0:26
- Biopolymers Essential for All Known Forms of Life That Are Composed of Nucleotides0:27
- Nucleotides Are Composed of These1:17
- Nucleic Acids Are Bound Inside Cells2:10
- Nitrogen Bases2:49
- Purines3:01
- Adenine3:10
- Guanine3:20
- Pyrimidines3:54
- Cytosine4:25
- Thymine4:33
- Uracil4:42
- Pentoses6:23
- Ribose6:45
- 2' Deoxyribose6:59
- Nucleotides8:43
- Nucleoside8:56
- Nucleotide9:16
- Example 110:23
- Polynucleotide Chains12:18
- What RNA and DNA Are Composed of12:37
- Hydrogen Bonding in DNA Structure13:55
- Ribose and 2! Deoxyribose14:14
- DNA Grooves14:28
- Major Groove14:46
- Minor Groove15:00
- Example 215:20
- Properties of DNA24:15
- Antiparallel Orientation24:25
- Phosphodiester Linkage24:50
- Phosphate and Hydroxyl Group25:05
- Purine Bases Always Pairs Pyramidine Bases25:30
- A, B, Z Forms25:55
- Major and Minor Grooves26:24
- Hydrogen Bonding and Hydrophobic Interactions Hold Strands Together26:34
- DNA Topology - Linking Number27:14
- Linking Number27:31
- Twist27:57
- Writhe28:31
- DNA Topology - Supercoiling31:50
- Example 333:16
Section 3: Maintenance of the Genome
Genome Organization: Chromatin & Nucleosomes
57m 2s
- Intro0:00
- Lesson Overview0:09
- Quick Glossary0:24
- DNA0:29
- Gene0:34
- Nucleosome0:47
- Chromatin1:07
- Chromosome1:19
- Genome1:30
- Genome Organization1:38
- Physically Cellular Differences3:09
- Eukaryotes3:18
- Prokaryotes, Viruses, Proteins, Small Molecules, Atoms4:06
- Genome Variance4:27
- Humans4:52
- Junk DNA5:10
- Genes Compose Less Than 40% of DNA6:03
- Chart6:26
- Example 18:32
- Chromosome Variance - Size, Number, and Density10:27
- Chromosome10:47
- Graph of Human Chromosomes10:58
- Eukaryotic Cell Cycle12:07
- Requirements for Proper Chromosome Duplication and Segregation13:07
- Centromeres and Telomeres13:28
- Origins of Replication13:38
- Illustration: Chromosome13:44
- Chromosome Condensation15:52
- Naked DNA to Start16:00
- Beads on a String16:13
- Mitosis16:52
- Start with Two Different Chromosomes17:18
- Split Into Two Diploid Cells17:26
- Prophase17:42
- Prometaphase17:52
- Metaphase19:10
- Anaphase19:27
- Telophase20:11
- Cytokinesis20:31
- Cohesin and Condensis21:06
- Illustration: Cohesin and Condensis21:19
- Cohesin21:38
- Condensin21:43
- Illustration of What Happens21:50
- Cohesins27:23
- Loaded During Replication and Cleaved During Mitosis27:30
- Separase27:36
- Nucleosomes27:59
- Histone Core28:50
- Eight Histone Proteins28:57
- Octamer of Core Histones Picture29:14
- Chromosome Condensation via H130:59
- Allows Transition to Compact DNA31:09
- When Not in Mitosis31:37
- Histones Decrease Available Binding Sites32:38
- Histone Tails33:21
- Histone Code35:32
- Epigenetic Code35:56
- Phosphorylation36:45
- Acetylation36:57
- Methylation37:01
- Ubiquitnation37:04
- Example 238:48
- Nucleosome Assembly41:22
- Duplication of DNA Requires Duplication of Histones41:50
- Old Histones Are Recycled42:00
- Parental H3-H4 Tetramers Facilitate the Inheritance of Chromatin States44:04
- Example 346:00
- Chromatin Remodeling48:12
- Example 453:28
DNA Replication
1h 9m 55s
- Intro0:00
- Lesson Overview0:06
- Eukaryotic Cell Cycle0:50
- G1 Growth Phase0:57
- S Phase: DNA & Replication1:09
- G2 Growth Phase1:28
- Mitosis1:36
- Normal Human Cell Divides About Every 24 Hours1:40
- Eukaryotic DNA Replication2:04
- Watson and Crick2:05
- Specific Base Pairing2:37
- DNA Looked Like Tetrinucleotide2:55
- What DNA Looks Like Now3:18
- Eukaryotic DNA Replication - Initiation3:44
- Initiation of Replication3:53
- Primer Template Junction4:25
- Origin Recognition Complex7:00
- Complex of Proteins That Recognize the Proper DNA Sequence for Initiation of Replication7:35
- Prokaryotic Replication7:56
- Illustration8:54
- DNA Helicases (MCM 2-7)11:53
- Eukaryotic DNA Replication14:36
- Single-Stranded DNA Binding Proteins14:59
- Supercoils16:30
- Topoisomerases17:35
- Illustration with Helicase19:05
- Synthesis of the RNA Primer by DNA Polymerase Alpha20:21
- Subunit: Primase RNA Polymerase That Synthesizes the RNA Primer De Navo20:38
- Polymerase Alpha-DNA Polymerase21:01
- Illustration of Primase Function Catalyzed by DnaG in Prokaryotes21:22
- Recap24:02
- Eukaryotic DNA Replication - Leading Strand25:02
- Synthesized by DNA Polymerase Epsilon25:08
- Proof Reading25:26
- Processivity Increased by Association with PCNA25:47
- What is Processivity?26:19
- Illustration: Write It Out27:03
- The Lagging Strand/ Discontinuing Strand30:52
- Example 131:57
- Eukaryotic DNA Replication - Lagging Strand32:46
- Discontinuous32:55
- DNA Polymerase Delta33:15
- Okazaki Fragments33:36
- Illustration33:55
- Eukaryotic DNA Replication - Okazaki Fragment Processing38:26
- Illustration38:44
- When Does Okazaki Fragments Happen40:32
- Okazaki Fragments Processing40:41
- Illustration with Okazaki Fragments Process Happening41:13
- Example 247:42
- Example 349:20
- Telomeres56:01
- Region of Repetitive Nucleotide Sequences56:26
- Telomeres Act as Chromosome Caps by Binding Proteins57:42
- Telomeres and the End Replication Problem59:56
- Need to Use a Primer59:57
DNA Mutations & Repairs
1h 13m 8s
- Intro0:00
- Lesson Overview0:06
- Damage vs. Mutation0:40
- DNA Damage-Alteration of the Chemical Structure of DNA0:45
- DNA Mutation-Permanent Change of the Nucleotide Sequence1:01
- Insertions or Deletions (INDELS)1:22
- Classes of DNA Mutations1:50
- Spontaneous Mutations2:00
- Induced Mutations2:33
- Spontaneous Mutations3:21
- Tautomerism3:28
- Depurination4:09
- Deamination4:30
- Slippage5:44
- Induced Mutations - Causes6:17
- Chemicals6:24
- Radiation7:46
- Example 18:30
- DNA Mutations - Tobacco Smoke9:59
- Covalent Adduct Between DNA and Benzopyrene10:02
- Benzopyrene10:20
- DNA Mutations - UV Damage12:16
- Oxidative Damage from UVA12:30
- Thymidine Dimer12:34
- Example 213:33
- DNA Mutations - Diseases17:25
- DNA Repair18:28
- Mismatch Repair19:15
- How to Recognize Which is the Error: Recognize Parental Strand22:23
- Example 326:54
- DNA Repair32:45
- Damage Reversal32:46
- Base-Excision Repair (BER)34:31
- Example 436:09
- DNA Repair45:43
- Nucleotide Excision Repair (NER)45:48
- Nucleotide Excision Repair (NER) - E.coli47:51
- Nucleotide Excision Repair (NER) - Eukaryotes50:29
- Global Genome NER50:47
- Transcription Coupled NER51:01
- Comparing MMR and NER51:58
- Translesion Synthesis (TLS)54:40
- Not Really a DNA Repair Process, More of a Damage Tolerance Mechanism54:50
- Allows Replication Past DNA Lesions by Polymerase Switching55:20
- Uses Low Fidelity Polymerases56:27
- Steps of TLS57:47
- DNA Repair1:00:37
- Recombinational Repair1:00:54
- Caused By Ionizing Radiation1:00:59
- Repaired By Three Mechanisms1:01:16
- Form Rarely But Catastrophic If Not Repaired1:01:42
- Non-homologous End Joining Does Not Require Homology To Repair the DSB1:03:42
- Alternative End Joining1:05:07
- Homologous Recombination1:07:41
- Example 51:09:37
Homologous Recombination & Site-Specific Recombination of DNA
1h 14m 27s
- Intro0:00
- Lesson Overview0:16
- Homologous Recombination0:49
- Genetic Recombination in Which Nucleotide Sequences Are Exchanged Between Two Similar or Identical Molecules of DNA0:57
- Produces New Combinations of DNA Sequences During Meiosis1:13
- Used in Horizontal Gene Transfer1:19
- Non-Crossover Products1:48
- Repairs Double Strand Breaks During S/Gs2:08
- MRN Complex Binds to DNA3:17
- Prime Resection3:30
- Other Proteins Bind3:40
- Homology Searching and subsequent Strand Invasion by the Filament into DNA Duplex3:59
- Holliday Junction4:47
- DSBR and SDSA5:44
- Double-Strand Break Repair Pathway- Double Holliday Junction Model6:02
- DSBR Pathway is Unique6:11
- Converted Into Recombination Products by Endonucleases6:24
- Crossover6:39
- Example 17:01
- Example 28:48
- Double-Strand Break Repair Pathway- Synthesis Dependent Strand Annealing32:02
- Homologous Recombination via the SDSA Pathway32:20
- Results in Non-Crossover Products32:26
- Holliday Junction is Resolved via Branch Migration32:43
- Example 334:01
- Homologous Recombination - Single Strand Annealing42:36
- SSA Pathway of HR Repairs Double-Strand Breaks Between Two Repeat Sequences42:37
- Does Not Require a Separate Similar or Identical Molecule of DNA43:04
- Only Requires a Single DNA Duplex43:25
- Considered Mutagenic Since It Results in Large Deletions of DNA43:42
- Coated with RPA Protein43:58
- Rad52 Binds Each of the Repeated Sequences44:28
- Leftover Non-Homologous Flaps Are Cut Away44:37
- New DNA Synthesis Fills in Any Gaps44:46
- DNA Between the Repeats is Always Lost44:55
- Example 445:07
- Homologous Recombination - Break Induced Replication51:25
- BIR Pathway Repairs DSBs Encountered at Replication Forks51:34
- Exact Mechanisms of the BIR Pathway Remain Unclear51:49
- The BIR Pathway Can Also Help to Maintain the Length of Telomeres52:09
- Meiotic Recombination52:24
- Homologous Recombination is Required for Proper Chromosome Alignment and Segregation52:25
- Double HJs are Always Resolved as Crossovers52:42
- Illustration52:51
- Spo11 Makes a Targeted DSB at Recombination Hotspots56:30
- Resection by MRN Complex57:01
- Rad51 and Dmc1 Coat ssDNA and Promote Strand Invasion and Holliday Junction Formation57:04
- Holliday Junction Migration Can Result in Heteroduplex DNA Containing One or More Mismatches57:22
- Gene Conversion May Result in Non-Mendelian Segregation57:36
- Double-Strand Break Repair in Prokaryotes - RecBCD Pathway58:04
- RecBCD Binds to and Unwinds a Double Stranded DNA58:32
- Two Tail Results Anneal to Produce a Second ssDNA Loop58:55
- Chi Hotspot Sequence59:40
- Unwind Further to Produce Long 3 Prime with Chi Sequence59:54
- RecBCD Disassemble1:00:23
- RecA Promotes Strand Invasion - Homologous Duplex1:00:36
- Holliday Junction1:00:50
- Comparison of Prokaryotic and Eukaryotic Recombination1:01:49
- Site-Specific Recombination1:02:41
- Conservative Site-Specific Recombination1:03:10
- Transposition1:03:46
- Transposons1:04:12
- Transposases Cleave Both Ends of the Transposon in Original Site and Catalyze Integration Into a Random Target Site1:04:21
- Cut and Paste1:04:37
- Copy and Paste1:05:36
- More Than 40% of Entire Human Genome is Composed of Repeated Sequences1:06:15
- Example 51:07:14
Section 4: Gene Expression
Transcription
1h 19m 28s
- Intro0:00
- Lesson Overview0:07
- Eukaryotic Transcription0:27
- Process of Making RNA from DNA0:33
- First Step of Gene Expression0:50
- Three Step Process1:06
- Illustration of Transcription Bubble1:17
- Transcription Starting Site is +15:15
- Transcription Unit Extends From the Promoter to the Termination Region5:40
- Example 16:03
- Eukaryotic Transcription: Initiation14:27
- RNA Polymerase II Binds to TATA Box to Initiate RNA Synthesis14:34
- TATA Binding Protein Binds the TATA Box14:50
- TBP Associated Factors Bind15:01
- General Transcription Factors15:22
- Initiation Complex15:30
- Example 215:44
- Eukaryotic Transcription17:59
- Elongation18:07
- FACT (Protein Dimer)18:24
- Eukaryotic Transcription: Termination19:36
- Polyadenylation is Linked to Termination19:42
- Poly-A Signals Near the End of the pre-mRNA Recruit to Bind and Cleave mRNA20:00
- Mature mRNA20:27
- Dissociate from Template DNA Strand21:13
- Example 321:53
- Eukaryotic Transcription25:49
- RNA Polymerase I Transcribes a Single Gene That Encodes a Long rRNA Precursor26:14
- RNA Polymerase III Synthesizes tRNA, 5S rRNA, and Other Small ncRNA29:11
- Prokaryotic Transcription32:04
- Only One Multi-Subunit RNA Polymerase32:38
- Transcription and Translation Occurs Simultaneously33:41
- Prokaryotic Transcription - Initiation38:18
- Initial Binding Site38:33
- Pribnox Box38:42
- Prokaryotic Transcription - Elongation39:15
- Unwind Helix and Expand Replication Bubble39:19
- Synthesizes DNA39:35
- Sigma 70 Subunit is Released39:50
- Elongation Continues Until a Termination Sequence is Reached40:08
- Termination - Prokaryotes40:17
- Example 440:30
- Example 543:58
- Post-Transcriptional Modifications47:15
- Can Post Transcribe your rRNA, tRNA, mRNA47:28
- One Thing In Common47:38
- RNA Processing47:51
- Ribosomal RNA47:52
- Transfer RNA49:08
- Messenger RNA50:41
- RNA Processing - Capping52:09
- When Does Capping Occur52:20
- First RNA Processing Event52:30
- RNA Processing - Splicing53:00
- Process of Removing Introns and Rejoining Exons53:01
- Form Small Nuclear Ribonucleoproteins53:46
- Example 657:48
- Alternative Splicing1:00:06
- Regulatory Gene Expression Process1:00:27
- Example1:00:42
- Example 71:02:53
- Example 81:09:36
- RNA Editing1:11:06
- Guide RNAs1:11:25
- Deamination1:11:52
- Example 91:13:50
Translation
1h 15m 1s
- Intro0:00
- Lesson Overview0:06
- Linking Transcription to Translation0:39
- Making RNA from DNA0:40
- Occurs in Nucleus0:59
- Process of Synthesizing a Polypeptide from an mRNA Transcript1:09
- Codon1:43
- Overview of Translation4:54
- Ribosome Binding to an mRNA Searching for a START Codon5:02
- Charged tRNAs will Base Pair to mRNA via the Anticodon and Codon5:37
- Amino Acids Transferred and Linked to Peptide Bond6:08
- Spent tRNAs are Released6:31
- Process Continues Until a STOP Codon is Reached6:55
- Ribosome and Ribosomal Subunits7:55
- What Are Ribosomes?8:03
- Prokaryotes8:42
- Eukaryotes10:06
- Aminoacyl Site, Peptidyl tRNA Site, Empty Site10:51
- Major Steps of Translation11:35
- Charing of tRNA11:37
- Initiation12:48
- Elongation13:09
- Termination13:47
- “Charging” of tRNA14:35
- Aminoacyl-tRNA Synthetase14:36
- Class I16:40
- Class II16:52
- Important About This Reaction: It Is Highly Specific17:10
- ATP Energy is Required18:42
- Translation Initiation - Prokaryotes18:56
- Initiation Factor 3 Binds at the E-Site19:09
- Initiation Factor 1 Binds at the A-Site20:15
- Initiation Factor 2 and GTP Binds IF120:50
- 30S Subunit Associates with mRNA21:05
- N-Formyl-met-tRNA22:34
- Complete 30S Initiation Complex23:49
- IF3 Released and 50S Subunit Binds24:07
- IF1 and IF2 Released Yielding a Complete 70S Initiation Complex24:24
- Deformylase Removes Formyl Group24:45
- Example 125:11
- Translation Initiation - Eukaryotes29:35
- Small Subunit is Already Associated with the Initiation tRNA29:47
- Formation of 43S Pre-Initiation Complex30:02
- Circularization of mRNA by eIF431:05
- 48S Pre-Initiation Complex35:47
- Example 238:57
- Translation - Elongation44:00
- Charging, Initiation, Elongation, Termination All Happens Once44:14
- Incoming Charged tRNA Binds the Complementary Codon44:31
- Peptide Bond Formation45:06
- Translocation Occurs46:05
- tRNA Released46:51
- Example 347:11
- Translation - Termination55:26
- Release Factors Terminate Translation When Ribosomes Come to a Stop Codon55:38
- Release Factors Are Proteins, Not tRNAs, and Do Not Carry an Amino Acid55:50
- Class I Release Factors55:16
- Class II Release Factors57:03
- Example 457:40
- Review of Translation1:01:15
- Consequences of Altering the Genetic Code1:02:40
- Silent Mutations1:03:37
- Missense Mutations1:04:24
- Nonsense Mutations1:05:28
- Genetic Code1:06:40
- Consequences of Altering the Genetic Code1:07:43
- Frameshift Mutations1:07:55
- Sequence Example1:08:07
Section 5: Gene Regulation
Gene Regulation in Prokaryotes
45m 40s
- Intro0:00
- Lesson Overview0:08
- Gene Regulation0:50
- Transcriptional Regulation1:01
- Regulatory Proteins Control Gene Expression1:18
- Bacterial Operons-Lac1:58
- Operon2:02
- Lactose Operon in E. Coli2:31
- Example 13:33
- Lac Operon Genes7:19
- LacZ7:25
- LacY7:40
- LacA7:55
- LacI8:10
- Example 28:58
- Bacterial Operons-Trp17:47
- Purpose is to Produce Trptophan17:58
- Regulated at Initiation Step of Transcription18:04
- Five Genes18:07
- Derepressible18:11
- Example 318:32
- Bacteriophage Lambda28:11
- Virus That Infects E. Coli28:24
- Temperate Lifecycle28:33
- Example 430:34
- Regulation of Translation39:42
- Binding of RNA by Proteins Near the Ribosome- Binding Site of the RNA39:53
- Intramolecular Base Pairing of mRNA to Hide Ribosome Binding Site40:14
- Post-transcriptional Regulation of rRNA40:35
- Example 540:08
Gene Regulation in Eukaryotes
1h 6m 6s
- Intro0:00
- Lesson Overview0:06
- Eukaryotic Transcriptional Regulations0:18
- Transcription Factors0:25
- Insulator Protein0:55
- Example 11:44
- Locus Control Regions4:00
- Illustration4:06
- Long Range Regulatory Elements That Enhance Expressions of Linked Genes5:40
- Allows Order Transcription of Downstream Genes6:07
- (Ligand) Signal Transduction8:12
- Occurs When an Extracellular Signaling Molecule Activates a Specific Receptor Located on the Cell8:19
- Examples9:10
- N F Kappa B10:01
- Dimeric Protein That Controls Transcription10:02
- Ligands10:29
- Example 211:04
- JAK/ STAT Pathway13:19
- Turned on by a Cytokine13:23
- What is JAK13:34
- What is STAT13:58
- Illustration14:38
- Example 317:00
- Seven-Spanner Receptors20:49
- Illustration: What Is It21:01
- Ligand Binding That Is Activating a Process21:46
- How This Happens22:17
- Example 424:23
- Nuclear Receptor Proteins (NRPs)28:45
- Sense Steroid and Thyroid Hormones28:56
- Steroid Hormones Bind Cytoplasmic NRP Homodimer29:10
- Hormone Binds NRP Heterodimers Already Present in the Nucleus30:11
- Unbound Heterodimeric NRPs Can Cause Deacetylation of Lysines of Histone Tails30:54
- RNA Interference32:01
- RNA Induced Silencing Complex (RISC)32:39
- RNAi33:54
- RISC Pathway34:34
- Activated RISC Complex34:41
- Process34:55
- Example39:27
- Translational Regulation41:17
- Global Regulation41:37
- Competitive Binding of 5 Prime CAP of mRNA42:34
- Translation-Dependent Regulation44:56
- Nonsense Mediated mRNA Decay45:23
- Nonstop Mediated mRNA Decay46:17
- Epigenetics48:53
- Inherited Patterns of Gene Expression Resulting from Chromatin Alteration49:15
- Three Ways to Happen50:17
- DNA Sequence Does Not Act Alone in Passing Genetic Information to Future Generations50:30
- DNA Methylation50:57
- Occurs at CpG Sites Via DNA Methyltransferase Enzymes50:58
- CpG Islands Are Regions with a High Frequency of CpG Sites52:49
- Methylation of Multiple CpG Sites Silence Nearby Gene Transcription53:32
- DNA Methylation53:46
- Pattern Can Be Passed to Daughter Cells53:47
- Prevents SP1 Transcription Factors From Binding to CpG Island54:02
- MECP254:10
- Example 555:27
- Nucleosomes56:48
- Histone Core57:00
- Histone Protein57:03
- Chromosome Condensation Via J157:32
- Linker Histone H157:33
- Compact DNA57:37
- Histone Code57:54
- Post-translational Modifications of N-Terminal Histone Tails is Part of the Epigenetic Code57:55
- Phosphorylation, Acetylation, Methylation, Ubiquitination58:09
- Example 658:52
- Nucleosome Assembly59:13
- Duplication of DNA Requires Duplication of Histones by New Protein Synthesis59:14
- Old Histones are Recycled59:24
- Parental H3-H4 Tetramers58:57
- Example 71:00:05
- Chromatin Remodeling1:01:48
- Example 81:02:36
- Transcriptionally Repressed State1:02:45
- Acetylation of Histones1:02:54
- Polycomb Repressors1:03:19
- PRC2 Protein Complex1:03:38
- PRC1 Protein Complex1:04:02
- MLL Protein Complex1:04:09
Section 6: Biotechnology and Applications to Medicine
Basic Molecular Biology Research Techniques
1h 8m 41s
- Intro0:00
- Lesson Overview0:10
- Gel Electraophoresis0:31
- What is Gel Electraophoresis0:33
- Nucleic Acids0:50
- Gel Matrix1:41
- Topology2:18
- Example 12:50
- Restriction Endonucleases8:07
- Produced by Bacteria8:08
- Sequence Specific DNA Binding Proteins8:36
- Blunt or Overhanging Sticky Ends9:04
- Length Determines Approximate Cleavage Frequency10:30
- Cloning11:18
- What is Cloning11:29
- How It Works12:12
- Ampicillin Example12:55
- Example 213:19
- Creating a Genomic DNA Library19:33
- Library Prep19:35
- DNA is Cut to Appropriate Sizes and Ligated Into Vector20:04
- Cloning20:11
- Transform Bacteria20:19
- Total Collection Represents the Whole Genome20:29
- Polymerase Chain Reaction20:54
- Molecular Biology Technique to Amplify a Small Number of DNA Molecules to Millions of Copies21:04
- Automated Process Now21:22
- Taq Polymerase and Thermocycler21:38
- Molecular Requirements22:32
- Steps of PCR23:40
- Example 324:42
- Example 434:45
- Southern Blot35:25
- Detect DNA35:44
- How It Works35:50
- Western Blot37:13
- Detects Proteins of Interest37:14
- How It Works37:20
- Northern Blot39:08
- Detects an RNA Sequence of Interest39:09
- How It Works39:21
- Illustration Sample40:12
- Complementary DNA (cDNA) Synthesis41:18
- Complementary Synthesis41:19
- Isolate mRNA from Total RNA41:59
- Quantitative PCR (qPCR)44:14
- Technique for Quantifying the Amount of cDNA and mRNA Transcriptions44:29
- Measure of Gene Expression44:56
- Illustration of Read Out of qPCR Machine45:23
- Analysis of the Transcriptome-Micrarrays46:15
- Collection of All Transcripts in the Cell46:16
- Microarrays46:35
- Each Spot Represents a Gene47:20
- RNA Sequencing49:25
- DNA Sequencing50:08
- Sanger Sequencing50:21
- Dideoxynucleotides50:31
- Primer Annealed to a DNA Region of Interest51:50
- Additional Presence of a Small Proportion of a ddNTPs52:18
- Example52:49
- DNA Sequencing Gel53:13
- Four Different Reactions are Performed53:26
- Each Reaction is Run in a Lane of a Denaturing Polyacrylamide Gel53:34
- Example 553:54
- High Throughput DNA Sequencing57:51
- Dideoxy Sequencing Reactions Are Carried Out in Large Batches57:52
- Sequencing Reactions are Carried Out All Together in a Single Reaction58:26
- Molecules Separated Based on Size59:19
- DNA Molecules Cross a Laser Light59:30
- Assembling the Sequences1:00:38
- Genomes is Sequenced with 5-10x Coverage1:00:39
- Compare Genomes1:01:47
- Entered Into Database and the Rest is Computational1:02:02
- Overlapping Sequences are Ordered Into Contiguous Sequences1:02:17
- Example 61:03:25
- Example 71:05:27
Section 7: Ethics of Modern Science
Genome Editing, Synthetic Biology, & the Ethics of Modern Science
45m 6s
- Intro0:00
- Lesson Overview0:47
- Genome Editing1:37
- What is Genome Editing1:43
- How It Works2:03
- Four Families of Engineered Nucleases in Use2:25
- Example 13:03
- Gene Therapy9:37
- Delivery of Nucleic Acids Into a Patient’s Cells a Treatment for Disease9:38
- Timeline of Events10:30
- Example 211:03
- Gene Therapy12:37
- Ethical Questions12:38
- Genetic Engineering12:42
- Gene Doping13:10
- Synthetic Biology13:44
- Design and Manufacture of Biological Components That Do Not Exist in Nature13:53
- First Synthetic Cell Example14:12
- Ethical Questions16:16
- Stem Cell Biology18:01
- Use Stem Cells to Treat or Prevent Diseases18:12
- Treatment Uses19:56
- Ethical Questions20:33
- Selected Topic of Ethical Debate21:30
- Research Ethics28:02
- Application of Fundamental Ethical Principles28:07
- Examples28:20
- Declaration of Helsinki28:33
- Basic Principles of the Declaration of Helsinki28:57
- Utmost Importance: Respect for the Patient29:04
- Researcher’s Duty is Solely to the Patient or Volunteer29:32
- Incompetent Research Participant30:09
- Right Vs Wrong30:29
- Ethics32:40
- Dolly the Sheep32:46
- Ethical Questions33:59
- Rational Reasoning and Justification35:08
- Example 335:17
- Example 438:00
- Questions to Ponder39:35
- How to Answer40:52
- Do Your Own Research41:00
- Difficult for People Outside the Scientific Community41:42
- Many People Disagree Because They Do Not Understand42:32
- Media Cannot Be Expected to Understand Published Scientific Data on Their Own42:43
Loading...
This is a quick preview of the lesson. For full access, please Log In or Sign up.
For more information, please see full course syllabus of Molecular Biology
For more information, please see full course syllabus of Molecular Biology
Molecular Biology DNA Replication
Bookmark & Share
Embed
Share this knowledge with your friends!
Copy & Paste this embed code into your website’s HTML
Please ensure that your website editor is in text mode when you paste the code.(In Wordpress, the mode button is on the top right corner.)
×
Since this lesson is not free, only the preview will appear on your website.
- - Allow users to view the embedded video in full-size.
Next Lecture
Previous Lecture
1 answer
Wed Jun 1, 2016 11:45 AM
Post by peter alabi on April 10, 2016
GOD, you're amazing you should totally teach genetics.
1 answer
Mon Feb 1, 2016 12:38 PM
Post by Luis Gallardo on January 3, 2016
Hi first of all, thanks for the great lecture! I have 2 questions though
1. Before the S phase...do we have chromosomes? or is it just chromatin lying around ...some more condensed than other (therefore, euchromatin and heterochromatin)..but the real condensing occurs in prophase and therefore formation of chromosomes (please correct me if Iam wrong)
2. I didn't quite understand the ligase function...so if the Pol Delta goes under the primer made by the Pol a and then continues on making more base pairs ...how is the backbone broken? if it supposedly only goes under the primer and automatically binds the next base pairs and then goes under the next primer when it encounters it again, (it will practically work like a leading chain) then I don't see how the ligase is needed at all.
Thank you very much in advance!
0 answers
Post by Professor Michael Philips on December 1, 2015
Glad you liked it!
1 answer
Tue Dec 1, 2015 11:26 PM
Post by Jaclyn Roland-McGowan on October 24, 2015
You are my life savor! Thank you for going into such detail!